INTRO MICROBES I
by Filipe Natalio
What are biominerals? What is biomineralisation? Why is it so important to study biominerals and biomineralisation? Can one use this knowledge towards applications with improved properties compared to current state-of-the art technologies?
Biomineralisation is a field of research that deals with the fabrication of minerals by biological systems. But it is much more than a simple “deconvolution” of the word biomineralisation. It is a biological process of accretion of atoms up to a macroscopic level which we then call a biomineral. This process is called either crystallization or polymerization, depending on the chemical nature of the atoms. However, this accretion of atoms can also be driven by physicochemical forces without the intervention of biological systems. These we call minerals and their chemistry is fairly well understood. Minerals such as calcite form abundantly in nature (e.g. found as chalk formations and used to produce cement). The atoms that compose a calcite (mineral) are exactly the same as the ones that compose calcite (biomineral) found in some shells, i.e., calcium (Ca2+) and carbonate (CO32-) ions. So, what is the difference between a calcite-based mineral and calcite-based biomineral? Here is where the most fundamental question of biomineralisation lies.
Let me start by giving several examples of biominerals. The most famous is bone, the second shells, then radiolarians and diatoms and finally and probably less well known, sponge spicules and calcium carbonate-producing bacteria. We are familiar with the concept that bones, in general, are tough and yet flexible (well at least to a certain extent). So, the first question is: if they are composed of the same atoms as the mineral carbonated hydroxylapatite (calcium phosphate with a carbonate group), what makes bones different from their mineral counterpart that is tough and brittle? First, bone is composed of very small particles (in the range of nanometers = 10^-9 meters) and not one single crystal as we find in minerals. Second, because it contains organic material between these very small particles that acts as “glue”. This soft “glue”, in turn provides two functionalities, one is to keep these very small particles together and the other is to provide flexibility. Biominerals are composed of one inorganic part (the mineral) and the organic part that fulfills numerous biological purposes (and bring evolutionary advantages) and have superior and improved properties (e.g. mechanical and/or light guiding) when compared to their pure inorganic counterparts. This premise holds true for all biominerals.
Both components of biomineral formation as well as its biochemistry and cell biology have been researched intensively. For example, it has been shown that the orientation of these hydroxylapatite nanoparticles in different bones is highly correlated to the forces exerted on them. Let’s consider a femur bone during walking. The femur is fixed to the coccyx with a certain angle. When we walk, and in each step we take, we are exerting a force on the femur that is almost four times our own weight. The force has to be distributed as homogenously as possible to avoid stress concentration and fracturing. Nature does this by placing each “nano”brick in a different orientation along the bone. One major breakthrough in the field of biomineralisation relates to the biochemistry and cell biology of this biological process. It was found that the inorganic nanobuilding blocks (carbonated hydroxylapatite in the case of bone) are formed inside specialized cells (osteoblasts in the case of bone) as amorphous precursors before forming the mineral. This seems to be a common mechanism underlying all biomineralising organisms. In the case of bones, the osteoblasts are mobile and unload their cargo at a particular site to either form a new bone or for regeneration purposes. In the case of diatoms, it is a single cell that does all the work of producing the siliceous frustule. In sponges, the specialized cells that produce the spicules are called sclerocytes.
The organic components of biominerals are normally found in very small amounts (only a few percent of the total weight) but, nonetheless, these amounts are able to radically change the properties of a bone from brittle to flexible. The organic material entrapped between the “nano”bricks is composed of biomolecules that can be simpler (e.g., polymeric chains of amines in diatoms) or more complex (e.g., proteins as found in sponge spicules). They can be divided into structural biomolecules (water insoluble fraction) and functional biomolecules (water soluble fraction). Simply put, the structural molecules act as a scaffolding for the deposition of mineral “nano”bricks while the functional molecules determine the accretion of the atoms in terms of size and shape. To sum up, these biomolecules play an important role in the overall biomineralisation process by displaying a wide array of functionalities (molecular backbone, templating/directing effect, nucleation and growth control) that are far from being fully understood.
Finally, I believe that this basic knowledge can be used in a wide variety of fields, and up to certain point, even replace current products and potentially overthrow current paradigms. For example, single cell radiolarians with incredibly complex shapes composed of silica were initially described by Ernst Haeckel. Radiolarians have inspired architects and engineers ever since the publication of Haeckel’s book, for example the geodesic domes of Buckminster Fuller.
Another example is siliceous spicules from deep-sea sponges. These rods are highly flexible and composed of glassy multilayers with different refractive indexes and, consequently, show a high total internal reflection of light. These glassy rods display remarkable fibre optical features, transmitting light more efficiently than the best optical fibres currently available on the market. Understanding this deep sea biomineral could lead to design more efficient light waveguides. Another example relates to the possibility of exploring the three-dimensional distribution of force lines and organic material in a bone during a dynamic process (e.g. walking) to design new hip implants based on the structure rather than the material and respecting the “biology”. Finally, calcium carbonate-producing bacteria under basic conditions – a process called microbially-induced calcium carbonate precipitation. These soil bacterial strains have been used predominantly in self-repairing concrete, soil amendment in mining sites and fabrication of masonry and architectural concepts.
Understanding the mechanisms of biomineral formation can yield fundamental knowledge about the complexity of a certain class of biological systems (biomineralising organisms) but it also holds a huge potential to serve as a platform to implement new biomimetic strategies towards a sustainable future that relies on bioinspired fabrication methodologies and products.
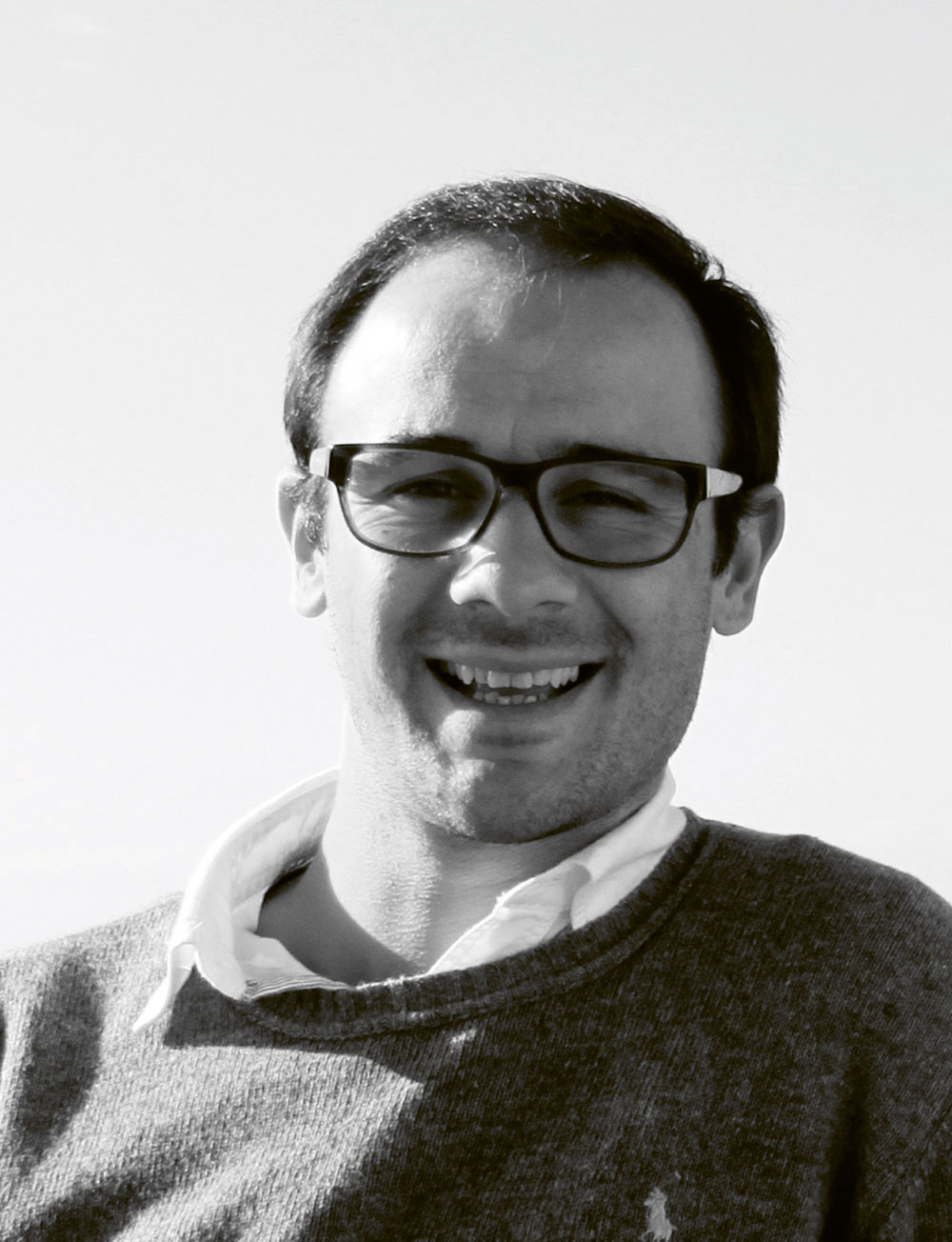
Dr. Filipe Natalio currently works as a scientist at the Kimmel Center for Archaeological Science that is part of the Weizmann Institute of Science in Rehovot, Israel. His formal training is in chemistry and biochemistry. His research has, however, led him into diverse fields that include biogenic silica, bionanotechnology, biomimetic catalysis, plant biology and archaeology.
We collaborated closely with Filipe Natalio during the project microbes I.